高分子94级校友、美国北卡罗莱纳大学化学系副教授尤为明天下午三点来我们系做报告,尤为博士毕业芝加哥大学,斯坦福大学博士后,2006到被卡大学化学系。他在高分子合成化学特别是高分子太阳能转换材料研究方面取得很好的研究称成果, 在JACS, Angew Chem Adv Mater 发表多篇论文,最近,诺贝尔化学奖获得者HEEGER在Science发表Highlight文章,高度评价了尤为教授的研究成果。邀请各位老师参加尤为的报告会,并通知感兴趣的研究生参加尤为的报告。 晚上六点在大雅楼信德厅系里的老师和尤为交流交流。
题目:Molecular Engineering of Conjugated Polymers to Reach Higher Efficiency "Plastic" Solar Cells
时间:2011年5月13日 15:00 (星期五下午三点)
地址:材料楼五楼会议室
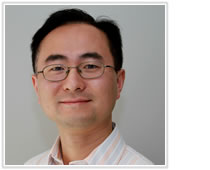
Assistant Professor
919-962-6197
919-962-2388 (fax)
Kenan C548
Group Page:http://www.chem.unc.edu/people/faculty/you/group/index.html
Organic and Polymer Synthesis, Organic Solar Cells, Molecular Electronics, Organic Spintronics
B.S. Chemistry, University of Science and Technology of China (1999); Ph.D. Organic/Polymer Chemistry, University of Chicago (2004); Postdoctoral Fellow, Stanford University (2004-2006); Tanner Award for Excellence in Undergraduate Teaching, 2011; Camille Dreyfus Teacher-Scholar Award, 2011
The You Group focuses on the synthesis and characterization of novel multifunctional materials for a variety of applications, predominately in electronics and photonics. Our approaches are truly interdisciplinary, interfacing chemistry, physics, materials science and engineering. Students and postdoctoral fellows in the group are exposed to and trained in organic and polymer synthesis, surface chemistry, nano-patterning, device fabrication, and physical properties characterization using state-of-the-art instrumentation.
Listed below are the three main research directions we are currently pursuing:
Organic Solar Cells
As the primary energy source for modern civilization (85% of total energy consumption), low cost fossil-fuels could be depleted as early as 2030. They also constitute the largest contributor to global warming. To reduce the greenhouse effect associated with burning fossil fuels and to sustain healthy growth of the world economy, a successful search for alternative, renewable energy sources is imperative. The most abundant and least polluting energy source by far is sunlight. This has led to widespread efforts to construct solar cells, i.e., photovoltaic (PV) cells, with high energy conversion efficiency and low production cost.
Currently, mainstream PV cells are inorganic semiconductors, mostly crystalline silicon. The energy conversion efficiency of these PV cells can reach 25%; however, the high cost of installed capacity (currently ~$4/W) severely restricts their large-scale application and makes them unattractive in most energy production markets. On the other hand, organic semiconductors offer a number of advantages for PV applications: (1) high optical absorption coefficients; (2) adjustable band gap to harvest a large fraction of the solar spectrum; (3) compatibility with flexible substrates; and (4) fabrication via low cost, high throughput printing techniques. What they currently lack, however, is adequate efficiency and lifetime.
A number of approaches to construct organic (or organic/inorganic hybrid) PV cells have been proposed and implemented, including dye-sensitized solid-state heterojunction cells, multilayer devices with stacked small molecule organic solar cells, donor-acceptor organic bulk heterojunction cells, organic-nanocrystal hybrid solar cells, and ordered organic-inorganic bulk heterojunction cells. However, at present, the highest energy conversion efficiency of organic PV cells is only 5 ~ 6%.
We are applying the following strategies to improve the conversion efficiency: (1) building well ordered materials/structures to capture more excitons and separate them more efficiently into mobile charges; (2) synthesizing small bandgap materials to harvest more photons; (3) engineering the interfaces between dissimilar materials to improve the charge transport; and (4) inducing/controlling assembly to enhance charge mobilities.
By applying novel multifunctional materials and conceptually new device designs, we expect to push the efficiency toward 10% and beyond. Low cost, highly efficient next generation solar cells will contribute greatly to a future renewable energy economy.
Molecular Electronics
Molecular electronics can be broadly defined as technologies utilizing single molecules, molecular assemblies, carbon nanotubes, or other nanoscale metallic or semiconductor wires to perform electronic functions. The rapid progress in shrinking the size of electronic components, as correlated by Moore's law, suggests that the ultimate in miniaturization will be constructing electronic circuits at the molecular or atomic level. The first theoretical model of molecular electronics was demonstrated by Aviram and Ratner, who proposed that individual molecules of the donor-spacer-acceptor (D-s-A) type between two electrodes would behave as molecular rectifiers under an electrical bias voltage. Extensive research efforts in the last decade in the area of molecular electronics have confirmed this potential and numerous nanostructured materials with different electronic functions, such as molecular wires, rectifiers, switches, and transistors, have been prepared and characterized.
We are working on two aspects of this exciting field: (1) new molecules, such as transition metal complexes inserted between conjugated oligomers to impart new functionalities to the molecular electronic devices; (2) fabrication of molecular electronic devices via non-destructive methods such as nano-transfer printing (nTP), with ultimate goal to integrate molecules based devices into large scale, functional circuit.
Organic Spintronics
Conventional electronic devices are charge based: they ignore the spin properties of the charge carriers (electrons). The emerging field of spintronics (spin transport electronics or spin-based electronics) is one in which the spin degree of freedom (up or down) of the electrons is used in addition to or instead of the charge degree of freedom, and it is full of opportunities. Since spin can be manipulated at a faster speed and with less energy than charges, spin-based devices should have faster switching times and decreased electric power consumption than conventional semiconductor devices. Numerous materials have been proposed or implemented for spin-based multifunctional devices, including memory, spin valves, spin-transistors, and spin-light emitting diodes.
Most of the research on spintronics has been conducted using inorganic materials, for example, alloys of nickel, iron and cobalt as ferromagnetic materials and copper as a nonmagnetic conductor; only a few reports have tested organic materials as the conducting layer in spin valves, and none of them have been scaled down to the molecular level. Molecular-scale organic spintronics devices would offer excellent potential for miniaturization of spintronics devices in the semiconductor and microelectronics industries.
Advantages of organic molecules for spintronics include: (1) organic molecules are small - usually a few nanometers in length - but fully functional, as demonstrated by the burgeoning field of molecular electronics; (2) spin diffusion length can be as long as 200 nm in organic molecules (e.g., oligo(thiophene)s); (3) organic chemistry offers a much larger palette for fine-tuning electronic properties by tweaking the structure and functional groups; and (4) these molecules can often be easily manipulated through self-assembly, whereas inorganic-based devices require expensive vacuum deposition facilities.
We are constructing these molecules based organic spin valves through a layer-by-layer approach by sequential assembly of organic molecules on ferromagnetic surfaces, and then ferromagnetic nanoparticles. A few important issues need to be addressed, such as spin injection, spin transport and spin detection.
We believe these new concepts in materials and nanofabrication will revolutionize the field of spintronics and aid in the discovery of novel device physics and applications.
youwei.jpg